Professor Anne Ferguson-Smith
Explains why, despite juggling multiple roles and responsibilities, she will always have time to discuss genetics.
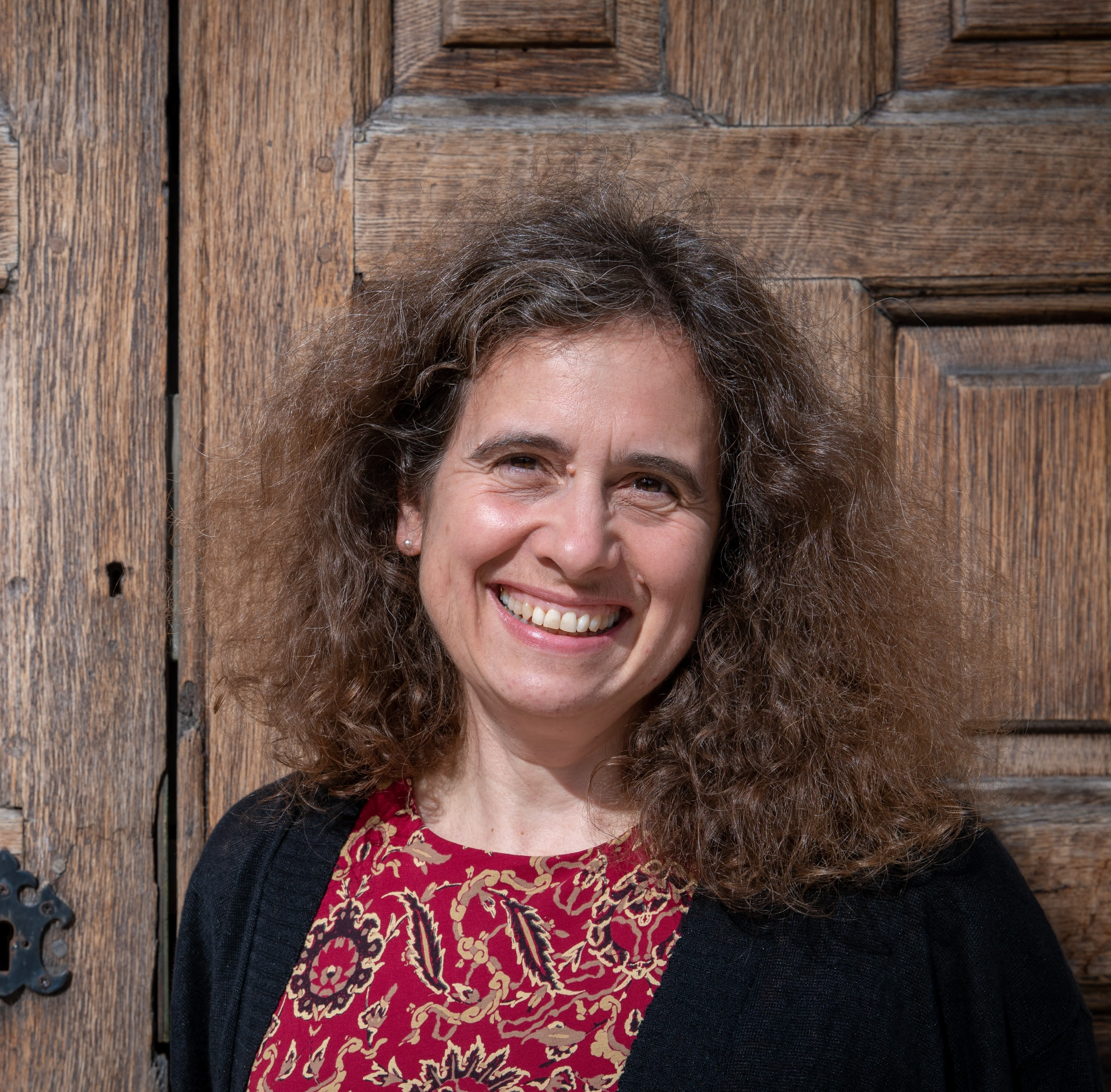
Professor Anne Ferguson-Smith is a busy woman. The Darwin Fellow and former Head of the University’s Department of Genetics is Arthur Balfour Professor of Genetics, Pro-Vice-Chancellor for Research and International Partnerships, Fellow of the Royal Society and President of the Genetics Society. The day we speak she is battling through jetlag, having returned from Singapore the day before. In June she was appointed CBE for services to Medical Research in the King’s Birthday Honours, and was Jim Al-Khalili’s guest on BBC Radio 4’s The Life Scientific.
Her role at the Genetics Society, which was founded in 1919 and promotes research, training, teaching and public engagement in all areas of genetics is “quite big and a real honour”, she says.
“I accepted the role before I knew I was going to become Pro-Vice-Chancellor, so my life turned out to be a little busier than I thought.”
However, you get the impression that if a job is connected to genetics, she would struggle to turn it down.
“I love genetics – I’m very happy to talk to people about genetics!” she says cheerfully.
As the daughter of medical geneticist and founder of the Cambridge Resource Centre for Comparative Genomics Professor Malcolm Ferguson-Smith, now aged 91, one could say she entered the family business. But Anne is dismissive of suggestions that she deliberately followed her father’s example.
“I had choices. I didn’t decide when I was young that I was going to be a scientist and then a geneticist, and I didn’t stand in awe of my father or want to follow in his footsteps. But I have always been fascinated by genetics and I did see my first chromosome when I was a child and was sort of smitten.”
However, while she plays down her own family’s contribution to it, Anne is rightly proud of the role that Cambridge as a whole has played in the development of her subject.
“The discovery of the structure of DNA happened 70 years ago, but before that there was a very strong history of genetics at Cambridge, starting from much earlier. I would say it was one of the birthplaces of genetics as a discipline. The Genetics Department was formed in 1912 with the endowment of the Balfour Professorship of Genetics, but before that William Bateson and colleagues such as Edith Saunders and Reginald Punnett (the first Balfour Professor), were working together and uncovering fundamental principles of genetics.”
This pride in Cambridge’s genetic credentials extends to the present. Anne is particularly clear that the University’s decision to retain a specific Department of Genetics, while other institutions are losing or combining them, was the right call.
“With the onset of genome biology and genome sequencing, the discipline of genetics was seen as a sort of tool that touched everything in the biological sciences. Now, of course, genome biology does touch most of biological and biomedical science, but the discipline of genetics, particularly in an educational context, is extremely important. It’s about the study of heredity and the study of inheritance, relates to evolution and populations and DNA is a central part of that. And there are multiple areas in which there are still plenty of conceptual advances to be made. So institutions that have lost their genetics departments I think are the poorer for it, particularly since our natural scientists and our medical students need to have a grounding in the understanding of inheritance in order to really effectively apply genome biology to what they do. All our students should have an understanding of genetics because of its impact on society.”
When Anne started out as a molecular geneticist, the focus of the field was on identifying and sequencing genes, as well as looking for mutations associated with particular inherited diseases. She points out that molecular genetic analysis has transformed both research and the analysis of patient populations.
“It’s an integral part now of early detection and prevention of disease, and it’s an integral part of our understanding of the complexities of our populations and how some people get diseases and others don’t. My particular area is the field of epigenetics. Of course, all our cells have the same DNA, and that DNA is packaged into these structures called chromosomes, and chromosome biology has been a longstanding field that’s very much depended on microscopy (cytogenetics). But now, with the advent of molecular genetics and sequencing technologies, the old classical type of cytogenetics of my father’s time doesn’t exist so much anymore, and molecular genetics and functional genomics is the direction of travel.”
As a leading authority on genomic imprinting and epigenetic mechanisms, Anne has devoted much of her career to expanding our understanding of how cell types can simultaneously have identical DNA and yet be different from each other.
“Epigenetics is in general about how DNA is packaged into chromosomes to influence both the structure of the chromosome and how genes are regulated along it,” she explains.
“So, for example, a brain cell packages its DNA in a way that enables genes that are important for brain function to be expressed, whereas a liver cell will package its chromosomes in such a way that those particular genes are not accessible in a liver cell, and instead liver genes are open for expression. Epigenetic modifications to DNA and to the proteins that package it, influence a gene’s accessibility to the factors that turn it on or off.
Epigenetic modifications are chemical marks that sit ‘on top’ of either the DNA or the histone proteins around which DNA is wrapped. One of the best studied is DNA methylation.”
Perhaps counterintuitively, however, epigenetic marks do not appear to pass between generations, something which Anne believes is frequently misunderstood.
“Genetic material, or DNA, is of course at the centre of inheritance and is transmitted from one generation to the next. But there’s a big question about the extent to which the epigenome can be transmitted from one generation to the next. You might imagine that if an epigenetic modification is sitting on the parent’s DNA, there is the potential for that non-genetic information to be transmitted to offspring. But actually one of the things that’s very important about epigenetic modifications in mammals is that the epigenome is erased and reconstructed, not once, but twice, from one generation to the next. Indeed, while a mammal is making the cells that are going to become the egg and the sperm, all the epigenetic marks are erased. So the primordial germ cell, the early cell that’s going to develop into an egg or a sperm, is epigenetically naked. As it divides and develops its identity, it acquires a new set of marks that are specific to it being an egg or a sperm. And then upon fertilisation those marks are mostly erased for a second time. So an epigenetic mark that is put on in one generation is removed, and then new epigenetic modifications are reconstructed as the new embryo develops. Although we don’t fully understand the details of this, I don’t think that concept is well appreciated.”
“Only a tiny proportion of our DNA encodes genes that code for proteins. But that doesn’t mean that the other 98% of it is not functionally relevant for example as regulatory sequences. In humans, around half of our DNA is repetitive - sequences of DNA that are repeated over and over and over again, and many of those sequences look like viral DNA.
“Many of those sequences also have the ability to jump around, because they contain some of those regulatory sequences and viral genes that enable them to integrate into the genome. It’s very important that is suppressed. One of the things that epigenetic modifications have evolved to do is to be targeted to those sequences and package them tightly to make them inaccessible to the gene expression machinery. Over time, some of these repetitive sequences have mutated, and now actually don’t really look like mobile elements anymore yet they are still epigenetically modified and act as regulatory sequences the behaviour of neighbouring genes. These sequences are not in identical places in different individuals, so they could explain why one individual is different from another or why one individual might have a disease and another one doesn’t.
The advent of CRISPR technology, enabling advances in the targeted editing of the genome, has transformed both experimental research in genetics and epigenetics, and the ability to develop therapeutic applications.”
Anne’s excitement and enthusiasm for the ever-evolving possibilities of genetic research are tangible, even as a hectic schedule across her multiple roles compete for her seemingly limitless energy. This year the Genetics Society marked the 70th anniversary of the structure of DNA with a series of lectures and prizes, celebrating a milestone which fixed genetics permanently in the public consciousness.
“I think we underestimate the public’s understanding of genetics; we found that especially after COVID, the public’s knowledge and interest is growing,” Anne says. “The public really care about the transmission of their own traits to their offspring and the impact of mutation and their lifestyles on themselves and their children. It’s a huge discipline, and the discovery of the structure of DNA was of course central to that, but there was a discipline before that and there remains plenty still to understand and apply in the future.”