Darwin and Sustainability
Solar-Driven Recycling of Waste into Fuel
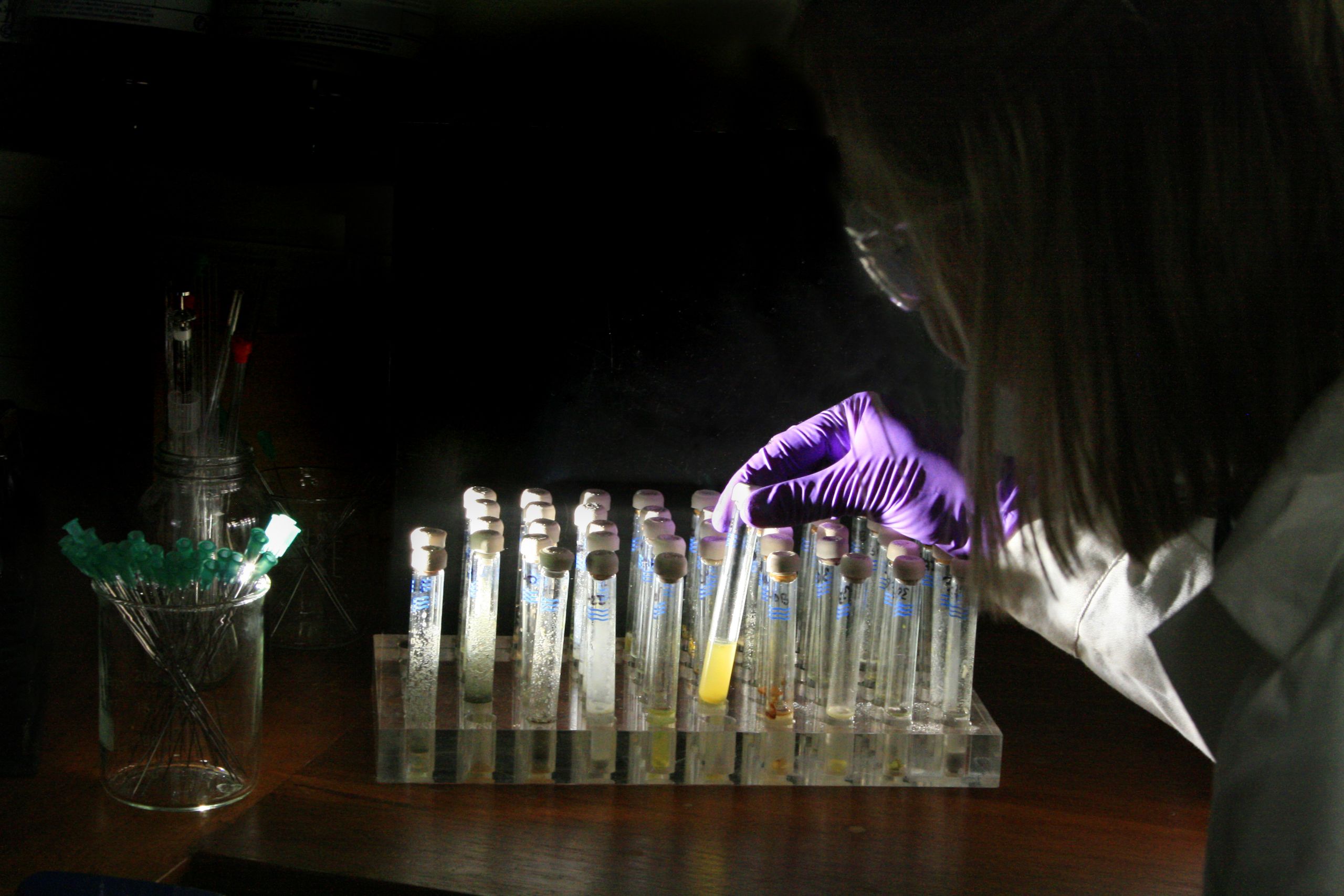
Dr Taylor Uekert (@TaylorUekert), Darwin alumna (matric: 2016) recently completed her PhD in the Reisner Lab as part of the Nanoscience & Nanotechnology Doctoral Training Centre and Darwin College. She will soon begin a postdoctoral position at the National Renewable Energy Laboratory (USA), where she will research circular economy strategies for photovoltaics and other renewable technologies.
Take a moment to look around yourself and think about what sort of plastic products you might throw away today. Perhaps there is some food packaging, or an old pen, or an empty soda bottle. These items quickly add up, and globally we produce 78 million tonnes of plastic packaging every year, or roughly how much eight thousand Eiffel towers weigh. With the advent of documentaries such as Blue Planet II, the enormous environmental impact of plastic waste has become increasingly apparent: 40% of all plastic packaging is landfilled and another 32% leaks directly into the environment. However, it may nevertheless come as a surprise that although 14% of all plastic packaging worldwide is collected for recycling, only 2% actually ends up being recycled back into the original product.
While much can be done in terms of reducing plastic consumption and developing alternative business models, new methods for recycling plastic waste into useful products are also essential, a topic being studied by Dr Taylor Uekert, a Darwin alumna who recently completed her PhD in the Reisner Lab at the Department of Chemistry. Taylor researches a process called photoreforming, which uses sunlight and a photocatalyst (a material that makes a chemical reaction happen faster) to simultaneously break down plastic waste and generate hydrogen (H2). H2 is a particularly interesting product given its many applications in the chemical and fertilizer industries, as well as its potential use as a clean fuel and heating source in the near future.
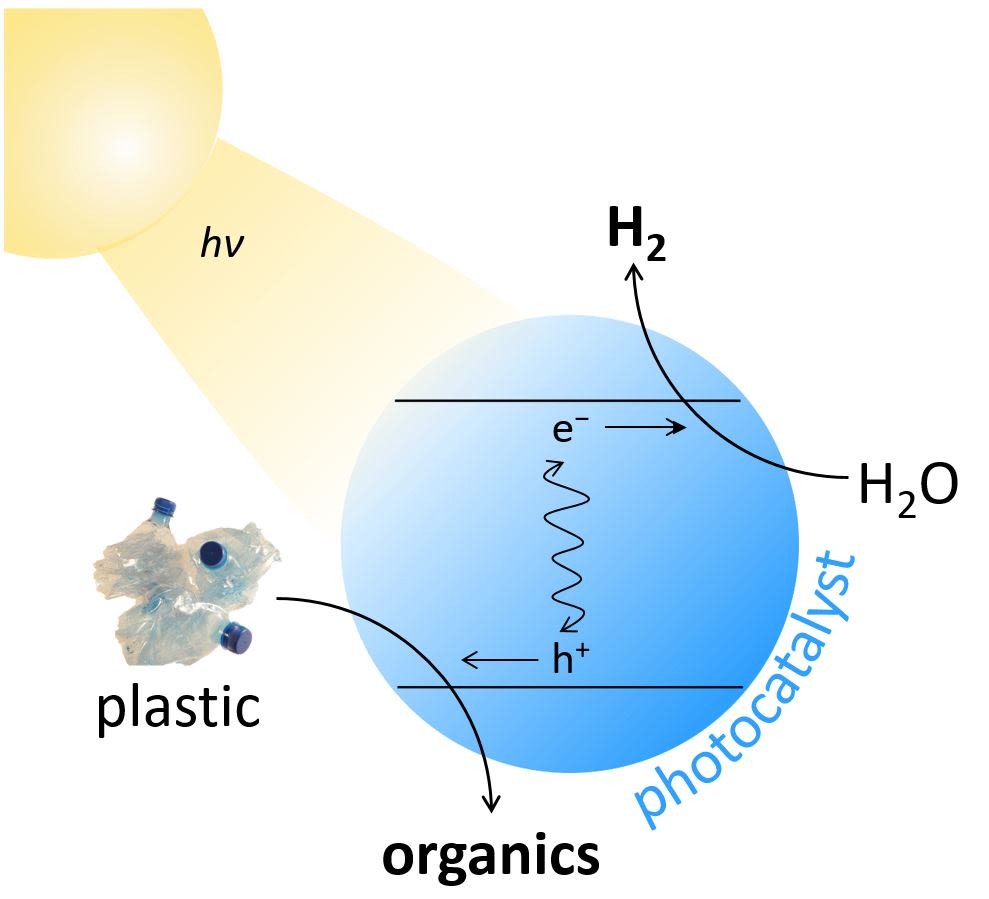
Schematic of the Photoreforming Process
Schematic of the Photoreforming Process
Photoreforming begins when sunlight shines upon a photocatalyst, which in Taylor’s case is an environmentally friendly material called carbon nitride coupled with nickel phosphide. That sunlight gives the electrons in the photocatalyst enough energy to break down water into H2 gas. The holes remaining in the photocatalyst then drive the breakdown of plastic into smaller organic molecules. These two chemical reactions occur simultaneously, thereby enabling not only the generation of a green fuel, but also the mitigation of waste that might be difficult to dispose of otherwise. Taylor and other researchers at the Reisner Lab have recently shown that photoreforming can produce H2 from common polar plastics such as polylactic acid (PLA), which is a biodegradable polymer commonly used in Vegware, and polyethylene terephthalate (PET), which is most commonly found in drink bottles and food packaging and accounts for 10% of global plastic waste. After one week of sunlight exposure, approximately 6-7% of these plastics can be converted into H2 and small, useful organic molecules such as formate and acetate. More importantly, photoreforming operates not just with pure plastics, but also with products that cannot be currently recycled. These include polyester microfibers, which are found in synthetic clothing and are too small to recycle, and oil-contaminated PET, which cannot be recycled due to its food contamination. Taylor has also looked beyond plastic alone and generated H2 from different types of food and mixed municipal waste, resulting in a process that is significantly more versatile than other recycling techniques.
In addition to demonstrating the wide scope of photoreforming, Taylor has also worked on immobilising the carbon nitride photocatalyst on a glass panel, which is important for up scaling and real-world processes in which immobilisation allows for facile photocatalyst recycling. The photocatalyst panels can produce H2 from a range of plastics and mixed waste and can be up scaled from a vial 1 cm in diameter to a flow reactor with an area of 25 cm2. The panels also enable H2 generation from dark, real-world waste that would otherwise prevent light from reaching the photocatalyst. For example, photoreforming run in this new flow reactor can still produce up to 50% as much H 2 under “realistic” conditions – 20% of the sunlight typically used in the lab (closer to UK irradiance) and seawater – as under “ideal” conditions (maximal sunlight and highly pure water). This is a crucial step for defining the overall sustainability and economic viability of a future commercial scale photoreforming setup.
While photoreforming benefits from its simplicity and versatility, just how “green” is it? To answer this question, Taylor and her co-workers modelled a hypothetical photoreforming pilot plant capable of handling between 100 and 300 kg of waste per day. They then calculated the carbon footprint, or how many greenhouse gases are emitted throughout the process chain per a certain amount of generated H2. With some improvements in efficiency, photoreforming is expected to produce H 2 with a lower environmental impact than existing waste-to-fuel technologies such as gasification or pyrolysis. Photoreforming also emits 40% greenhouse gases than disposing of waste in a landfill. From an economic perspective, there are still many things to improve, particularly photocatalyst efficiency and lifetime. However, all of these topics and more are currently being investigated in the Reisner Lab, with an aim to bring photoreforming to real-world application within the next ten years.
To learn more, please visit the Reisner Lab’s website and Twitter , or watch their informational videos.
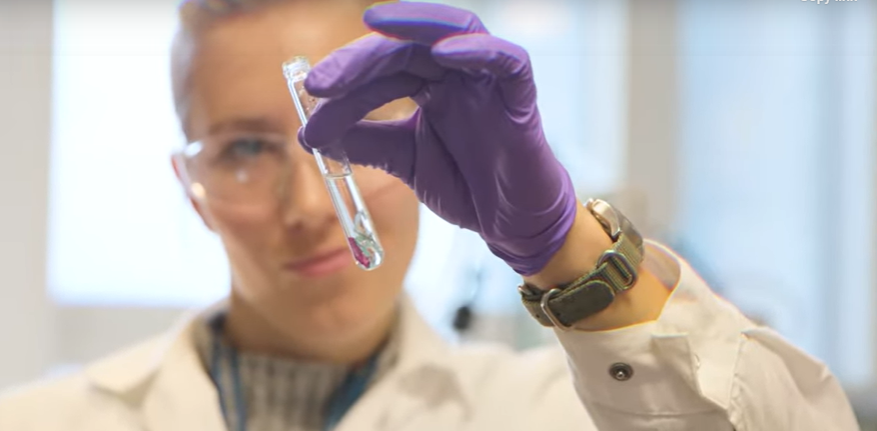
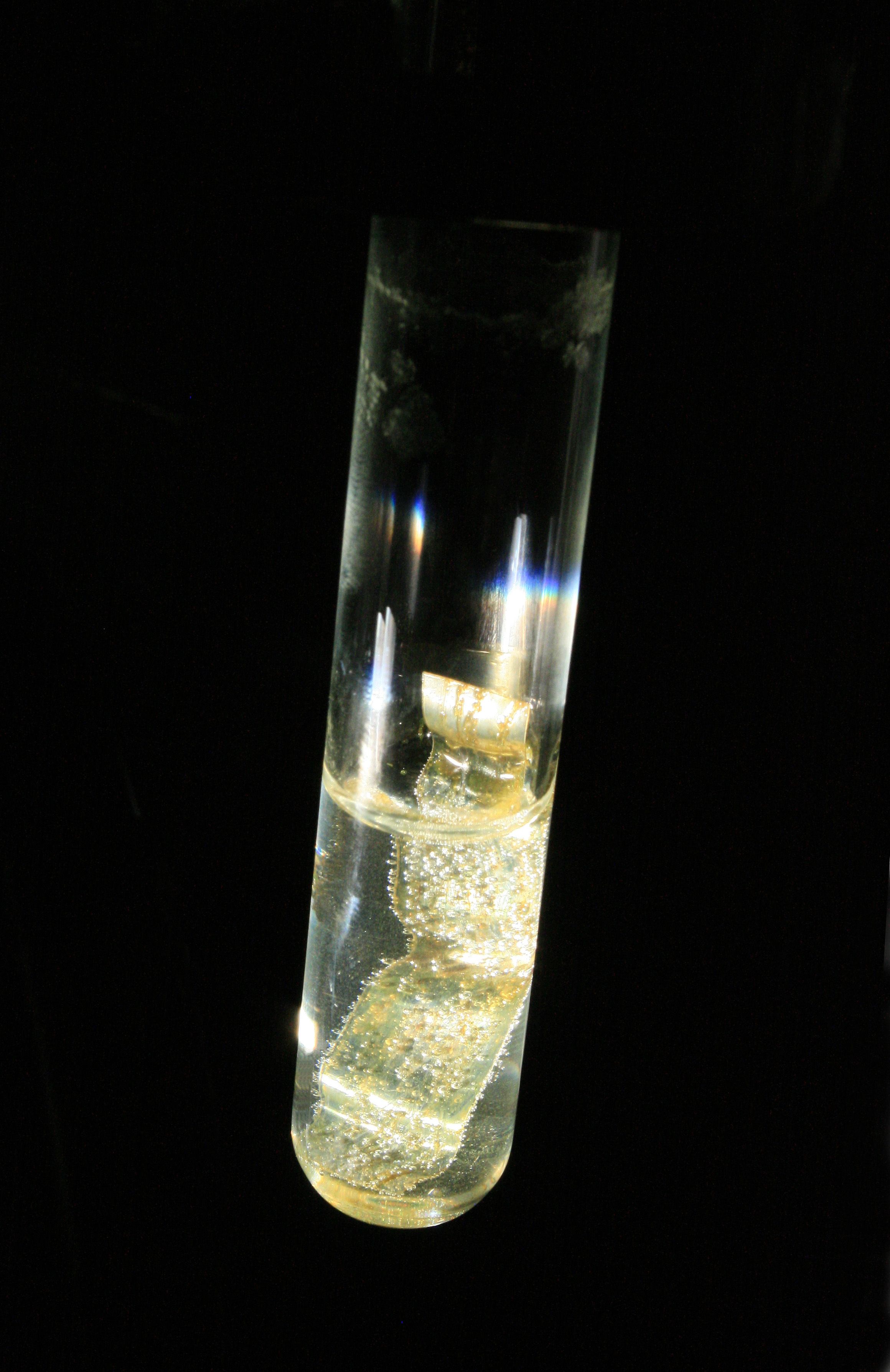
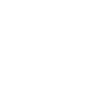